Our new project at GEOMAR!
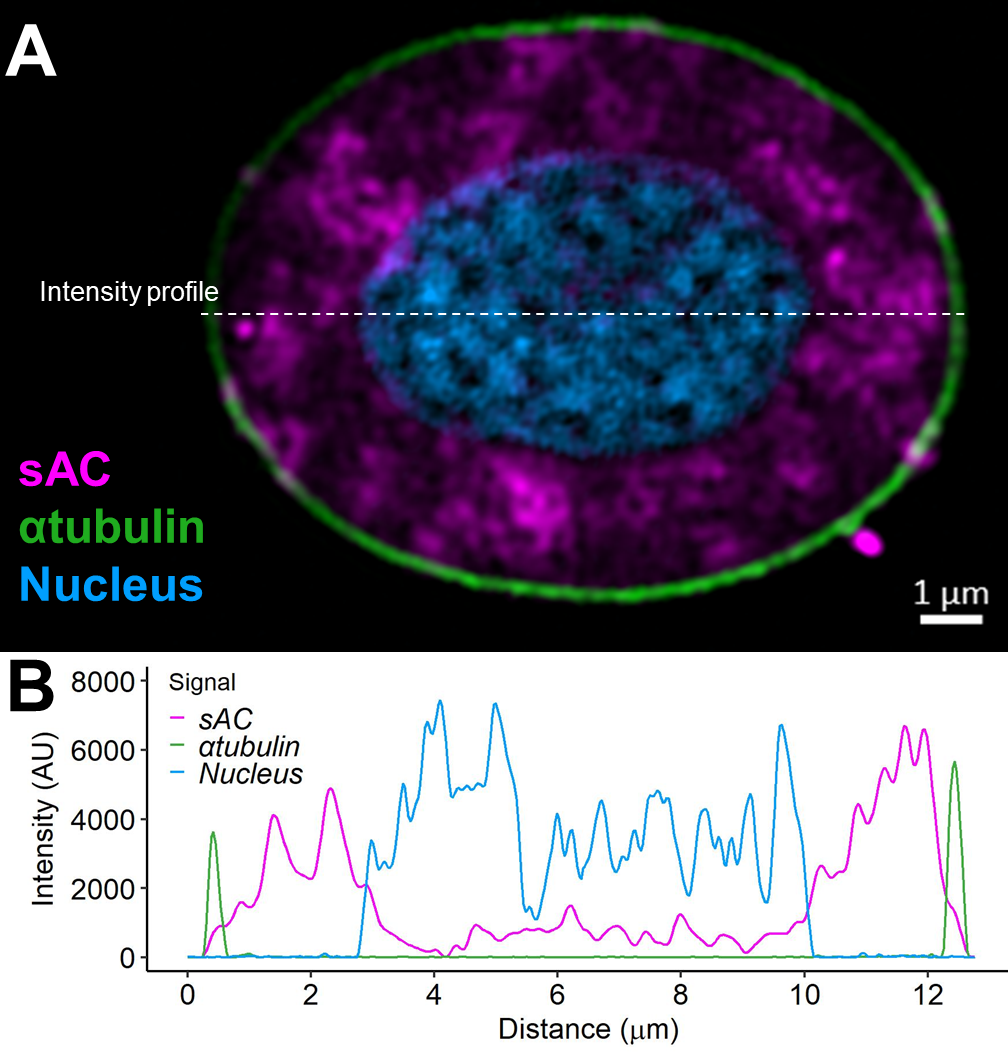
distribution of intracellular proteins in a rainbow
trout red blood cell.
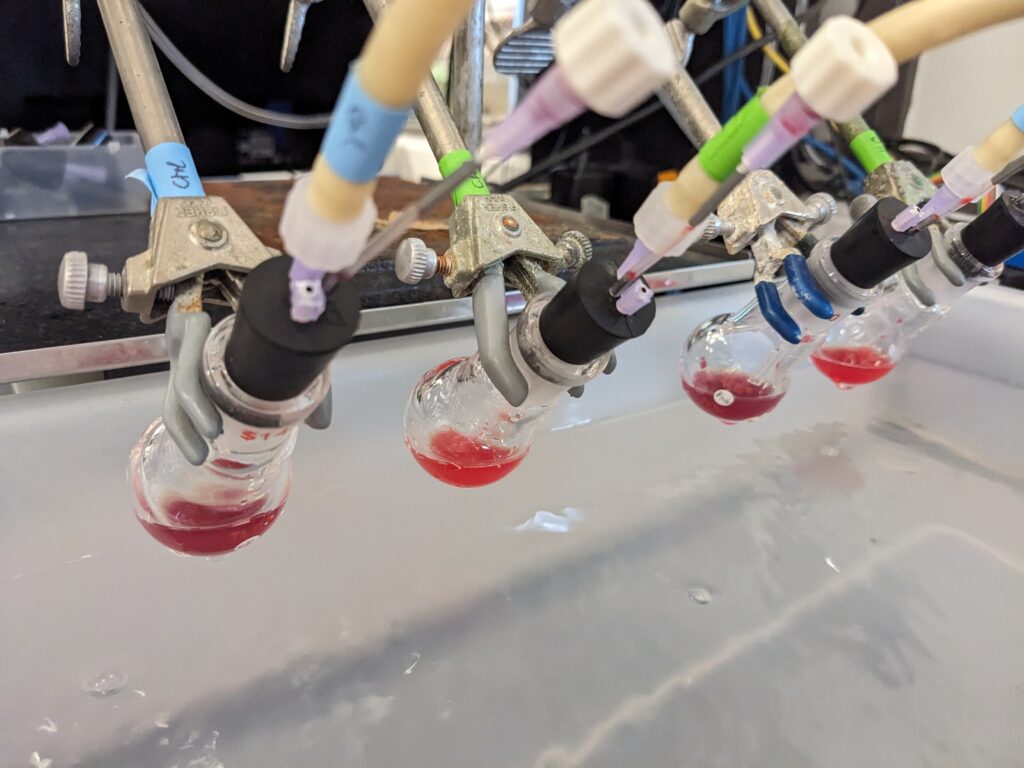
release of ATP from deer mouse red blood cells.
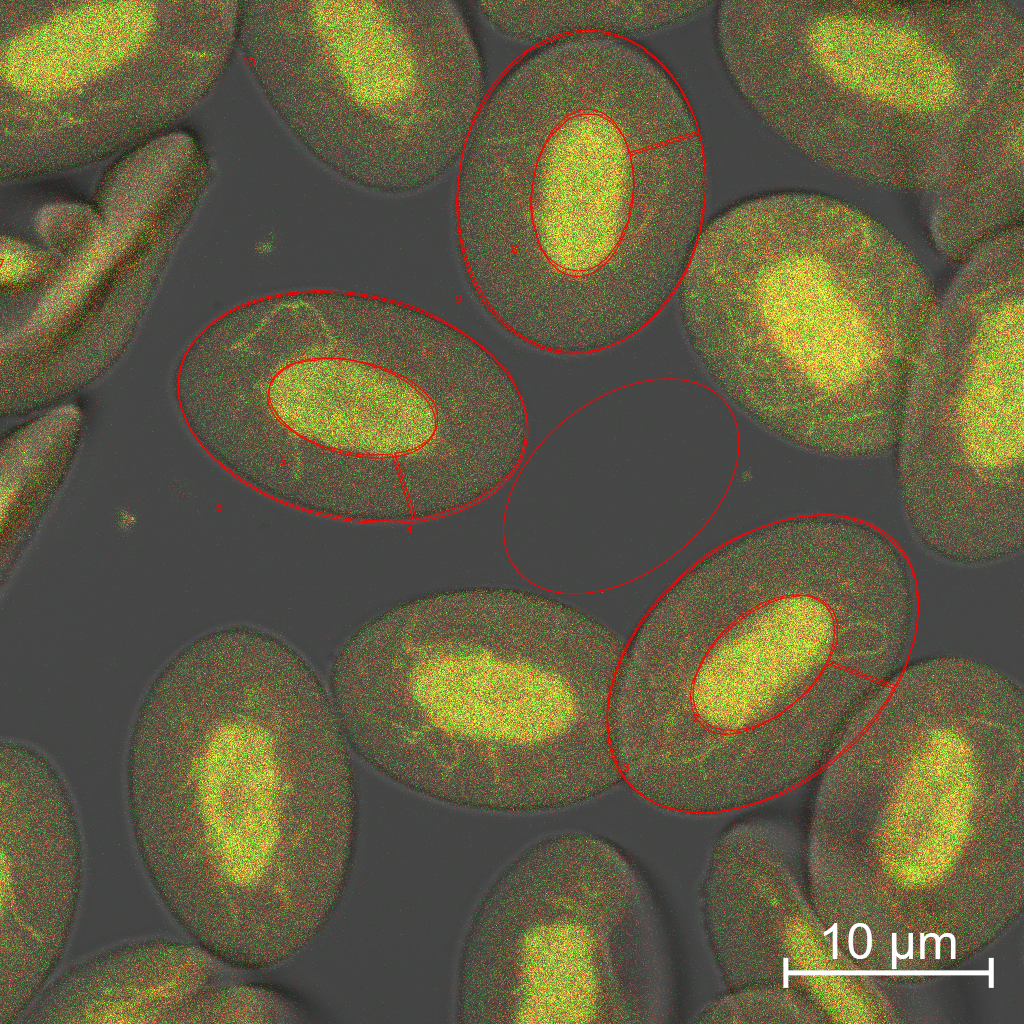
intracellular pH with the fluorophore SNARF1-AM.
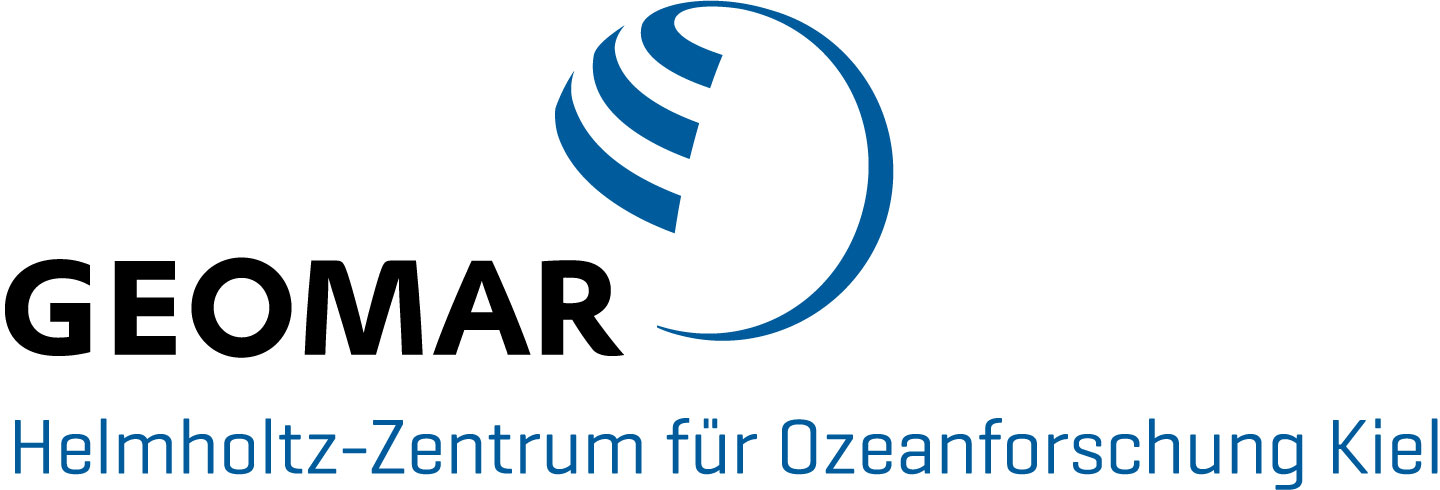
Comparative red blood cell physiology: uncovering novel cellular mechanisms to predict the impacts of climate change on fishes
Background | As global ocean temperatures increase, so does the body temperature of fishes, a group that represent half of all vertebrate species. The challenge is two-fold: warmer water contains less oxygen while warmer body temperatures increase oxygen demand. Overcoming this fundamental imbalance between oxygen supply and demand may be the key for fish species to survive in a changing world. But climate change is not simply a temperature problem: rising levels of atmospheric carbon dioxide acidify the water and the blood of fishes, and eutrophication depletes aquatic oxygen creating hypoxic “dead zones”. How fishes respond to these environmental stressors is still poorly understood, but the mechanisms that cause ecological change at the population and ecosystems levels will be based on physiological limitations in individuals. There is a critical need for experimental work at the cellular and molecular levels to advance our fundamental understanding of the mechanisms that drive ecological change in aquatic ecosystems. The bulk of oxygen transport from the environment to the tissues is carried out by red blood cells. However, surprisingly, the cellular responses of red blood cells to environmental stressors are still poorly understood.
Objective | To study the physiological mechanisms at the level of the red blood cell, by which fishes balance cardiovascular gas transport and metabolic demand over a broad range of environmental and metabolic conditions.
Model system | Populations of Atlantic cod (Gadus morhua) from the Baltic, Atlantic and Arctic Oceans are experiencing vastly different rates of environmental change and thus, present a unique opportunity to study local adaptations that help them overcome these stressors. The species is of great commercial interest for fisheries and aquaculture and maintains a pivotal ecological role as a top predator.
General approach | Atlantic cod will be caught in the wild using the research vessels at GEOMAR. Sampling of blood in the field will be used for genomic and transcriptomic studies to reveal signatures of adaptive changes in red blood cell function. In the lab, fish will be acclimated to climate change relevant conditions of warming, hypoxia and ocean acidification. Phenotypic plasticity in red blood cell function will be studied in vivo and in vitro, while whole animal performance will be assessed by respirometry and swimming trials. The combined approach will allow establishing functional links between red blood cell function and organismal performance in Atlantic cod. Other projects will study the cellular and molecular mechanisms of oxygen and acid-base sensing in red blood cells, the release of signalling molecules and their effect on downstream targets in the vasculature and the tissues.
Outcomes | By using an integrative approach across multiple levels of biological organisation this project will advance our fundamental understanding of how vertebrate red blood cells maintain the fine balance between cardiovascular gas transport and metabolic demand. This knowledge will be critical to understand, predict and mitigate the impacts of climate change on fishes, aquatic ecosystems and human economic activities that depend on them (aquaculture, fisheries, tourism).
Job Openings | We are hiring a postdoc and several PhD and MSc students for the different parts of this project (find out more).
A selection of past projects
For a complete list see Publications.
Linking functional red blood cell phenotypes to environmental tolerance in high-altitude adapted deer mice
High-altitude deer mice (Peromyscus maniculatus) have evolved a higher haemoglobin oxygen affinity and other coordinated changes across the oxygen transport cascade that enable higher metabolic rates in hypoxia. Red blood cells have well-establishes functions in transporting oxygen and carbon dioxide in the blood, but may also play a role in matching oxygen supply and demand in the microcirculation by releasing signaling molecules such as ATP and gasotransmitters. However, how these cellular mechanisms respond to hypoxic environments has not been studied. We hypothesized that high-altitude adaptation and/or hypoxia acclimation in the lab may improve the function of red blood cell for cardiovascular gas transport in hypoxia. Using a common-garden experimental design, deer mice from high- and lowland populations were acclimated to warm normoxia (21°C, 21 kPa O2) or cold hypobaric hypoxia (5°C, 12 kPa O2) for 8 weeks. This experimental design allowed to examine the interactive effects of environmentally-induced plasticity and evolutionary adaptation to cold hypoxic environments on red blood cell phenotype. Our recent results show that cold hypoxic acclimation induced significant changes in red blood cell abundance, size, gene expression (measured in the bone marrow), abundance of membrane proteins, carbonic anhydrase and anion exchanger (band 3) activities, hypoxic ATP signalling pathways, buffer capacity and haemoglobin oxygen binding characteristics. Stay tuned for the publication of the full data set!
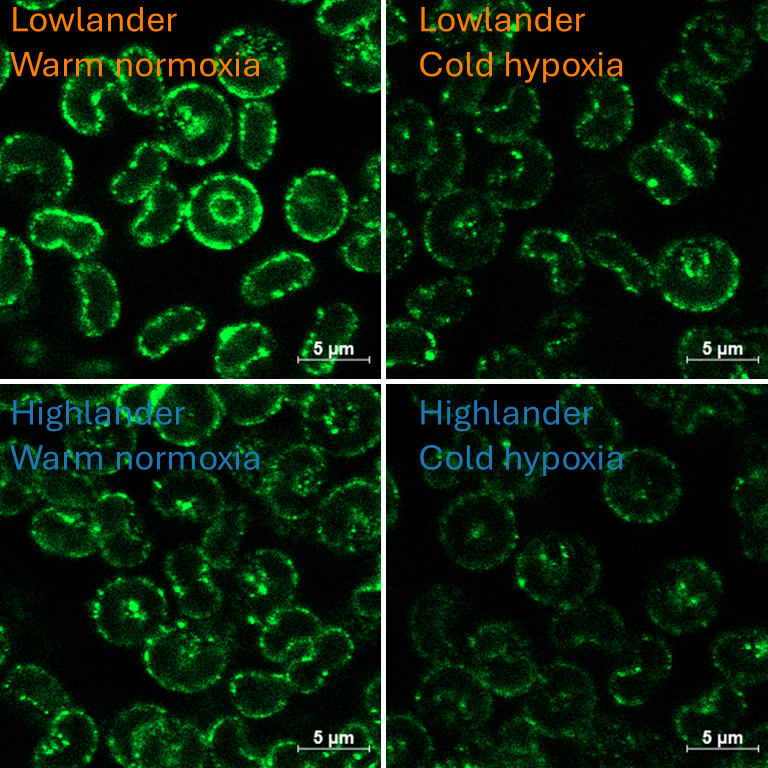
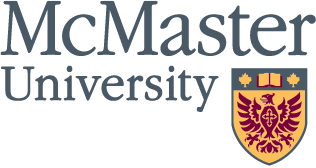
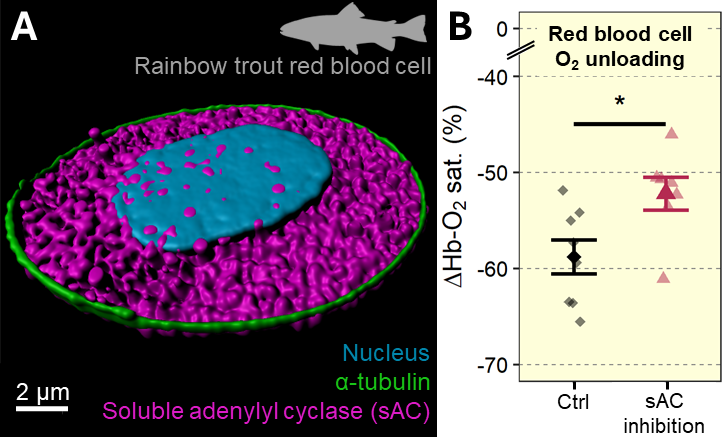
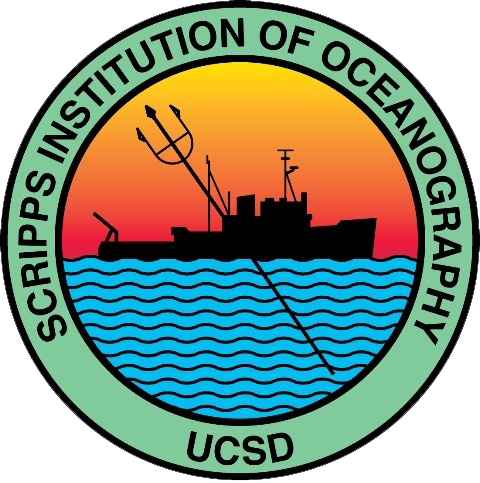
Red blood cells sense conditions in the vasculature to fine-tune oxygen unloading
Most vertebrates have pH-sensitive haemoglobins such that controlling red blood cell pH may be used to fine-tune cardiovascular oxygen transport. Recent work from our group found the novel bicarbonate-sensing molecule, soluble adenylyl cyclase (sAC) in the red blood cells of rainbow trout (read more). In a subsequent study we sought to identify the physiological function of this acid-base sensing enzyme, for red blood cell homeostasis and systemic oxygen transport. Using super-resolution microscopy we detected distinct pools of sAC protein in the red blood cell cytoplasm, at the plasma membrane and within the nucleus. Live-cell imaging of of red blood cell intracellular pH showed that sAC inhibition decreased the set point for cellular pH regulation and slowed the rates of pH recovery after an acid-base disturbance. During in vitro simulations of arterial-venous transit, sAC inhibition decreased the amount of oxygen that is unloaded from red blood cells by ~11%. Therefore, acid-base sensing by sAC represents a novel pathway in the red blood cells of rainbow trout, that participates in the regulation cellular pH and systemic blood oxygen transport (read more).
Fish red blood cells enhance oxygen unloading
Optimizing and enhancing aerobic metabolism has been central to the adaptive radiation of vertebrates. Prior to our investigation, a potential mechanism had been suggested that could greatly enhance oxygen unloading from pH-sensitive haemoglobin in teleost fishes, and that relies upon interactions between adrenergic sodium-proton exchangers (β-NHE) on the red blood cell membrane and carbonic anhydrase in the vasculature. Our work on Atlantic salmon provided the first in vivo evidence that this novel mechanism is a critical part of the cardiovascular oxygen transport pathway of teleosts. Our work revealed that teleost fishes, which represent half of all vertebrates, may enhance oxygen transport by an active cellular mechanism at the level of the red blood cell that responds to adrenergic control. The implications are important, as reproductive success in salmon depends on their ability to increase oxygen transport during up-river spawning migrations.
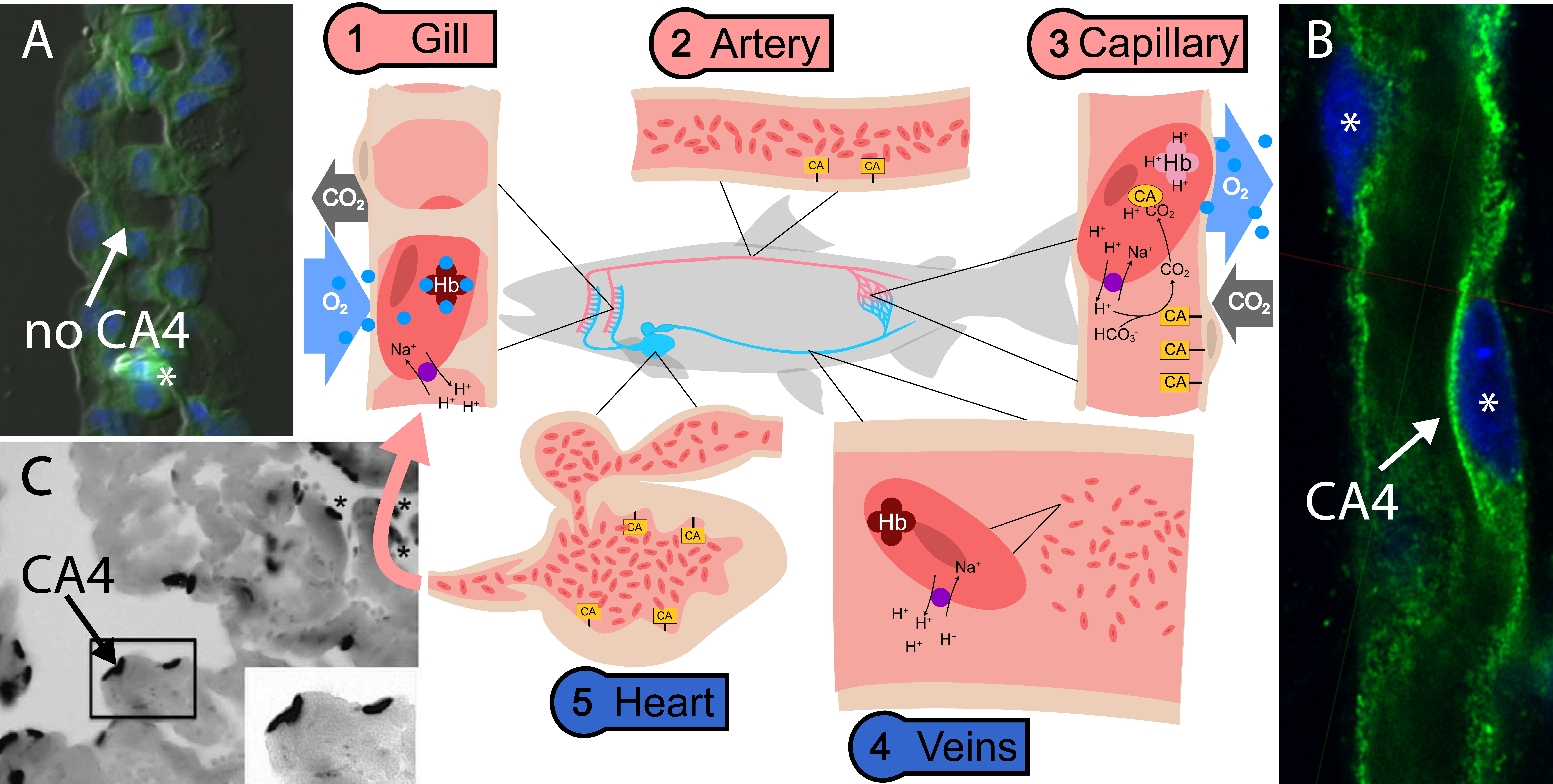
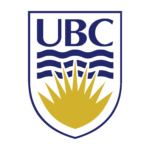
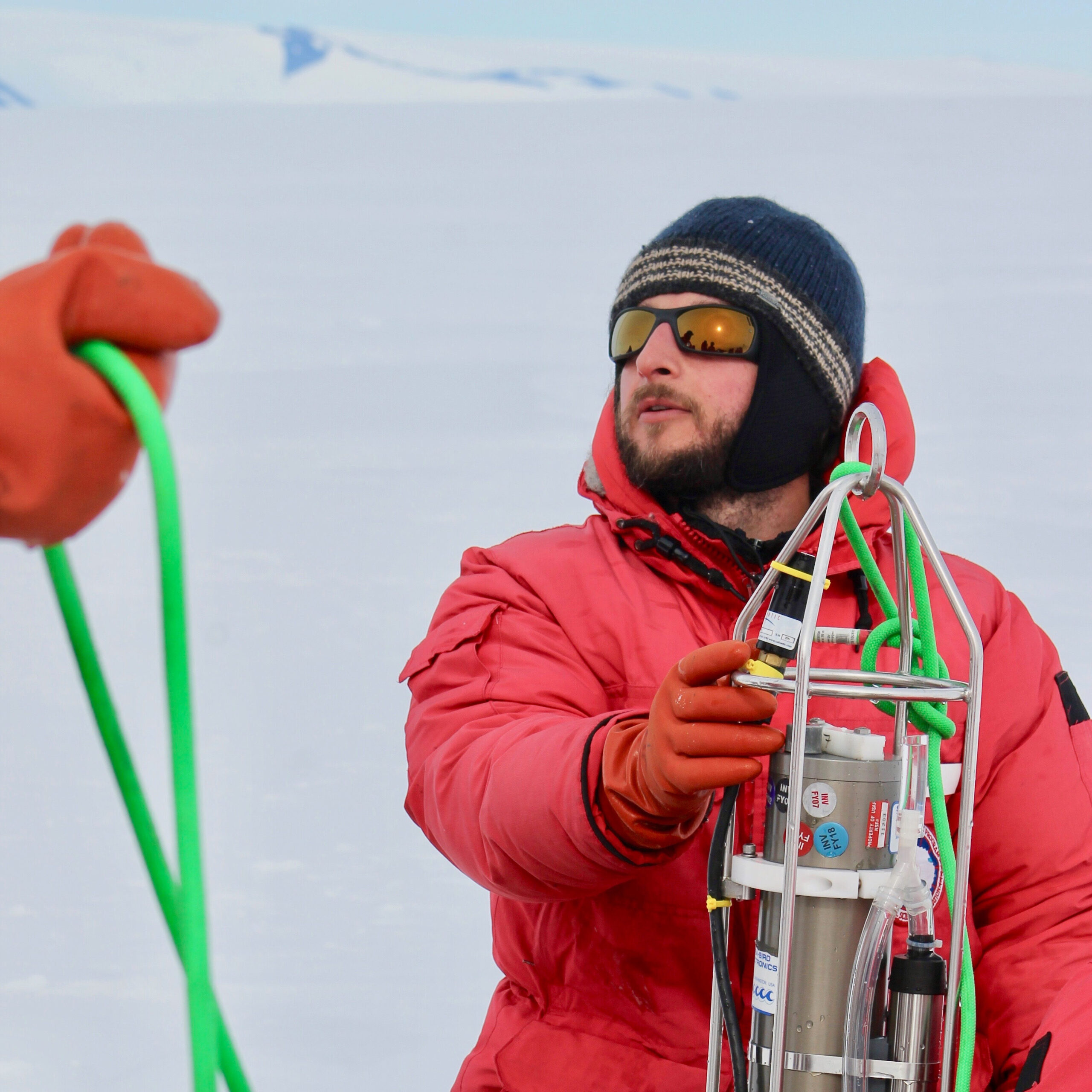
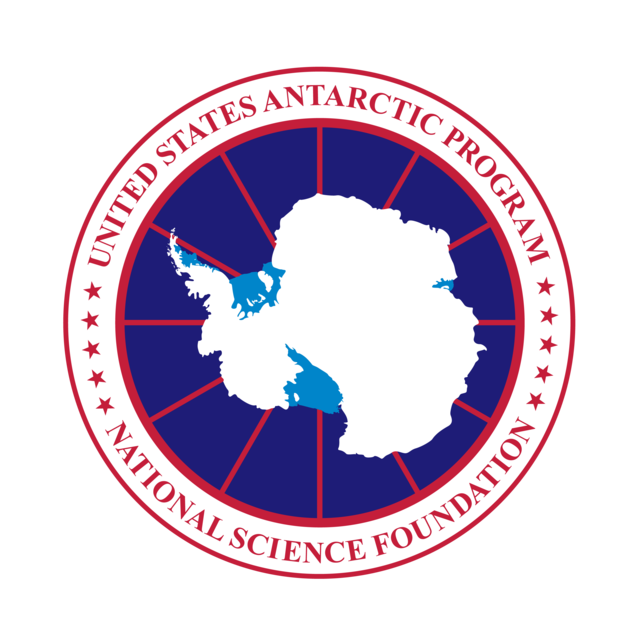
Antarctic icefish gills catalyze CO2 excretion
Antarctic icefish are the only known vertebrates to have lost red blood cells and haemoglobin from the circulation. Extreme adaptations in their cardiovascular physiology enable cardiovascular oxygen transport despite a ten-fold lower oxygen transport capacity of their blood. However, how icefishes catalize carbon dioxide excretion from the blood in the absence of red blood cell carbonic anhydrase, was still unknown. Our work showed that the gills of Antarctic icefishes express a membrane-bound carbonic anhydrase isoform that fully compensates for the loss of the enzyme within red blood cells. These findings revealed an exceptional evolutionary transition in the vertebrate CO2 excretion pathway and provide a framework to address more general questions on the evolutionary dynamics of vertebrate gas exchange pathways. The resulting publication (find it here) was awarded the JEB Outstanding Paper Prize in 2018.